Connecting plasma physics and engineering aspects in innovative technology design and fusion machine operation was the focus of the third IAEA technical meeting on divertor concepts, held in Vienna this week and attended by some 70 international experts. Nuclear fusion is the process that powers the sun and harnessed in a fusion reactor can provide a virtually unlimited supply of safe and carbon emission-free energy. A critical piece in its development is the so called divertor, which refers to the only part of the reactor where the extremely hot plasma in which the reaction takes place comes into direct contact with the reactor vessel.
Enabling Technology for Fusion: IAEA Forum Pushes Forward Advanced Mock-ups
The ITER tungsten divertor: the most sophisticated tokamak divertor ever built. 54 individual cassettes, fully water cooled, designed to handle up to 100 MW of fusion power. (Image: ITER Organization)
The meeting was a unique occasion to discuss divertor physics in various magnetic configurations, novel ideas for next step devices and, most importantly, the ITER divertor – the largest and most complex divertor ever constructed. Although this design reflects current understanding and technology, requirements to remove access power and impurities generated during the reaction from the plasma needs further development for future fusion power plants.
Inside a tokamak – the donut-shaped fusion device, a plasma is heated to hundreds of millions of degrees and confined by magnetic fields that keep it from the wall of the machine. The divertor is the ‘exhaust pipe’ target chamber located at the very bottom of the reactor, where impurities such as Helium ‘ash’ are diverted through outer magnetic field lines to a location far from the plasma.
This configuration produces ‘purer’ plasmas with better energy confinement – a critical parameter for the performance of a fusion device, thus maintaining the plasma hot enough for long enough, for fusion reactions to take place on a substantial scale, and at the same time, protecting the surrounding walls from thermal and neutronic loads.
In ITER – the international fusion reactor scale experiment being assembled in France – the divertor will be made up of 54 ten tons cassettes. These will need to be replaced by remote handling at least once during the machine's lifetime. Watch this video to see how remote handling tasks are carried out at JET, currently the world’s largest experimental fusion device.
Divertor geometries
The ITER divertor will face heat fluxes of 10-20 MW per square metre, ten times higher than the heat load on a spacecraft re-entering Earth's atmosphere! Its most exposed surface will face a maximum expected temperature between 1000 °C and 2000 °C. For this reason, and for its low tritium (fusion fuel) retention, Tungsten, a refractory metal that melts only at a very high temperature (3400 °C), has been chosen as the armour material for the components facing the plasma.
Future ITER operation experience will provide much of the technological basis for the design of future fusion power plants capable of generating electricity, including tests of divertor systems. Finding more resistant materials and the optimal magnetic configuration to better handle the heat fluxes at the divertor is a main focus of ongoing fusion research and development.
Designing divertor systems with pumps able to distinguish between Helium generated in fusion of Deuterium and Tritium (two Hydrogen isotopes) and unburned fusion fuel is another focus of ongoing research.
"The core plasma operational scenario imposes a number of constraints on the divertor design systems for reactor-scale tokamaks," said Anthony Leonard, scientist at the US General Atomics, and chair of the meeting.
"In the core we want high plasma density values to achieve high levels of fusion power and these need to be compatible with the values determined by the divertor geometry at the plasma edge," said Saskia Mordijck, an Assistant Professor at the US College William and Mary.
ITER and beyond
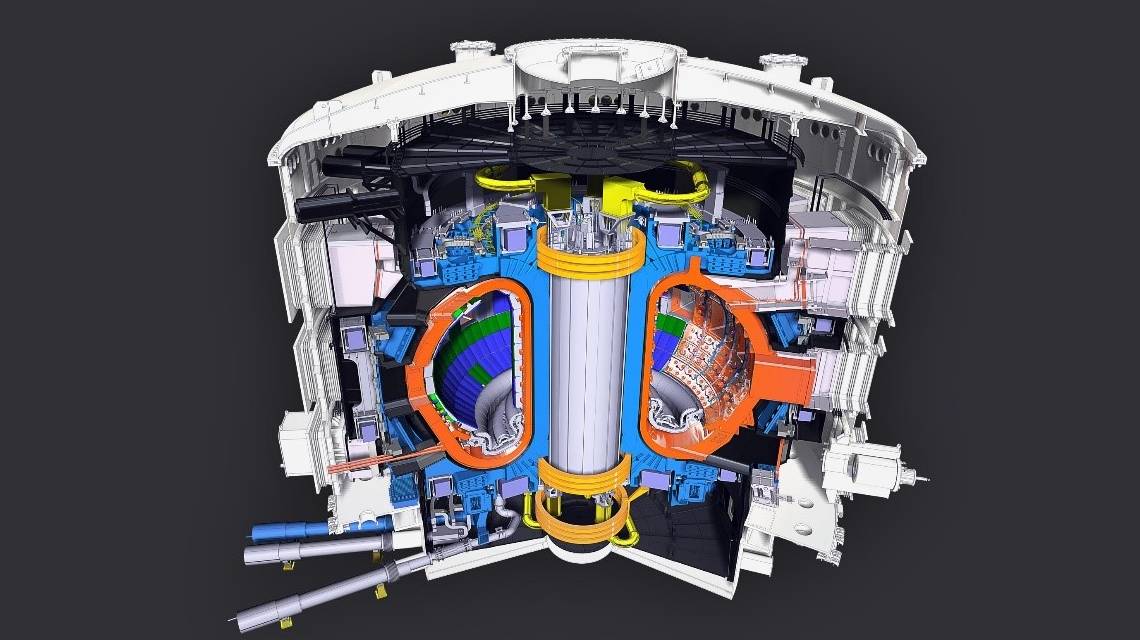
The ITER tokamak model: Divertor with its 54 cassettes (grey) are at the bottom of the vacuum vessel (orange). (Image: ITER Organization)
Richard Pitts, the ITER Organization’s Experiments and Plasma Operation Section Leader, focussed his presentation on the steady state operating regime of the ITER divertor, describing how advances in R&D since the divertor design began two decades ago have reinforced the need for operation at high neutral pressure in the plasma in the divertor region. He emphasised the need for a strong continuing boundary plasma physics and materials research programme in the next decade before ITER’s operation at high power.
This will be of relevance particularly for next generation experimental devices following ITER. These include DEMO, which are supposed to generate electricity, opening the way for a prototype fusion reactor. The IAEA is coordinating international roadmaps on the development of DEMO.
“Such R&D is of direct relevance to divertors in DEMO reactors, as will be operation of the ITER divertor itself since scale size is a key aspect of divertor performance,” Pitts said.
Meeting presenters included Nobuyuki Asakura, a senior scientist at the Japanese QST Naka Fusion Institute, who discussed divertor operation for Japanese DEMO. “Divertor heat removal is one of the most challenging issues on DEMO and conventional divertor design based on the ITER concept is being carried out in different approaches, depending on the main plasma performances. Our divertor code simulation for the Japanese power exhaust concept so far shows that the peak heat load can be handled by ITER divertor technology,” Asakura said.
For the European DEMO, conceptual studies of the divertor include assessments of alternative designs. Fulvio Militello, a scientist at the United Kingdom’s Atomic Energy Authority, discussed divertor solutions that cannot be tested at ITER. “In general, all alternative configurations are more complex than the ITER baseline Single Null design as far as the engineering is concerned. However, investigating the continuum between the standard and alternative solutions might lead to beneficial and acceptable designs,” Militello said.
Researchers in China have made significant progress in planning for a device called the Chinese Fusion Engineering Testing Reactor (CFETR), another device building on the experience of ITER. Rui Ding, a researcher at the Institute of Plasma Physics, Chinese Academy of Sciences, presented recent progress and possible solutions on divertor physics design of CFETR. “The CFETR divertor must meet requirements beyond that of ITER, and the divertor solution also needs to be compatible with high core fusion plasma performance, which means low impurity contamination and efficient impurity helium exhaust,” Ding said.
Starting a national research and technology programme leading to the construction of a compact pilot plant (CPP), which is supposed to produce electricity from fusion at the lowest possible capital cost, was one of the main recommendations of a recent US strategic fusion planning assessment. John Canik, Interim Director at the Fusion Energy Division of the US Oak Ridge National Laboratory, discussed a proposed 10-year programme aimed at demonstrating the combined physics and engineering basis for a divertor solution for a tokamak-based CPP. “Although there are important distinctions, many of the goals of a CPP are similar to other tokamak-based pathways like EU-DEMO and CFETR, which should allow for collaborative pursuit of power exhaust solutions,” Canik said.