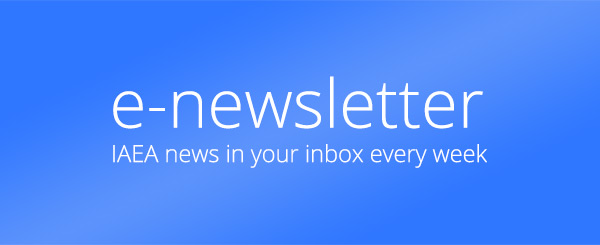
If you would like to learn more about the IAEA’s work, sign up for our weekly updates containing our most important news, multimedia and more.
Magnetic Fusion Confinement with Tokamaks and Stellarators
Wolfgang Picot
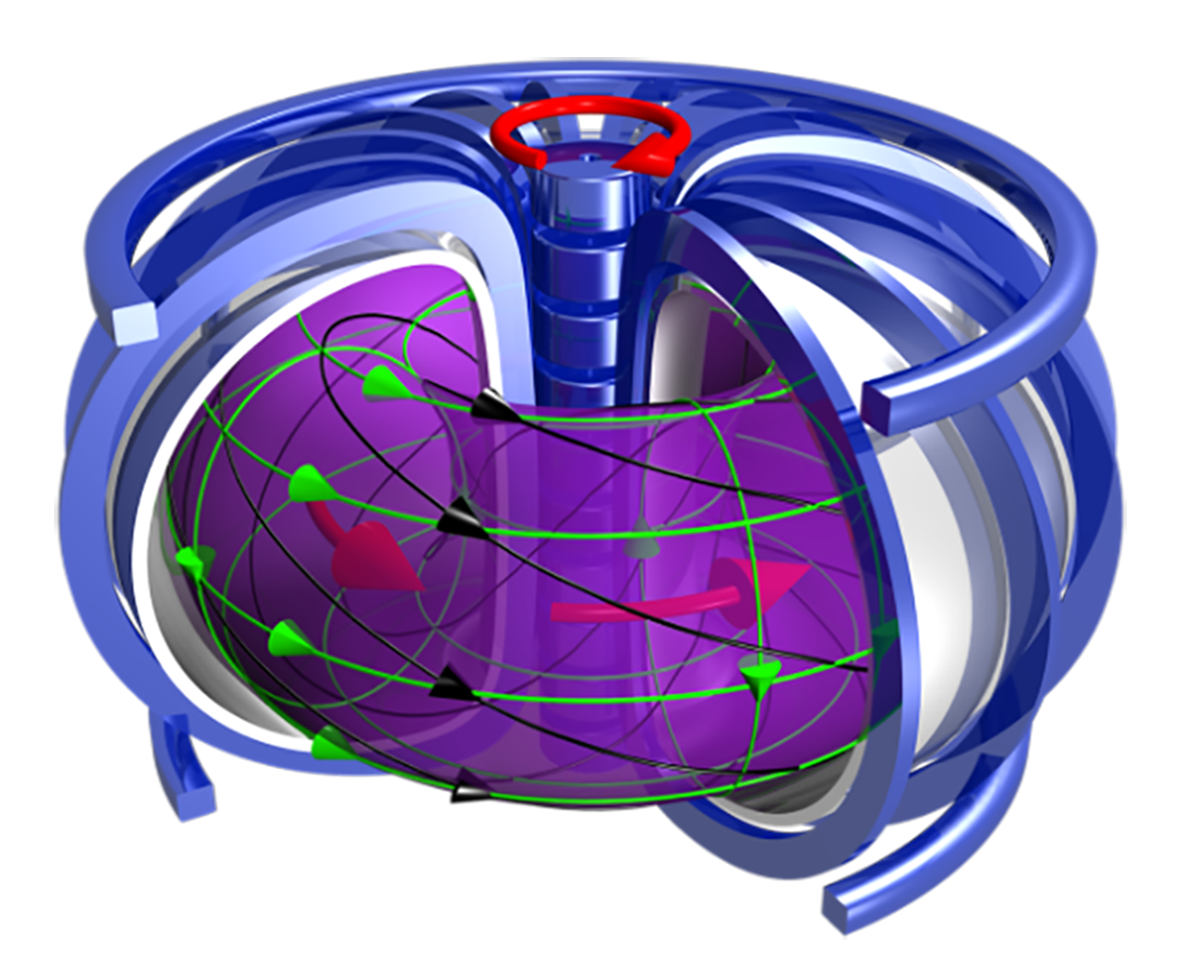
How a tokamak works: The electric field induced by a transformer drives a current (big red arrows) through the plasma column. This generates a poloidal magnetic field that bends the plasma current into a circle (green vertical circle). Bending the column into a circle prevents leakage and doing this inside a doughnut-shaped vessel creates a vacuum. The other magnetic field going around the length of the doughnut is referred to as toroidal (green horizontal circle). The combination of these two fields creates a three-dimensional curve, like a helix (shown in black), in which the plasma is highly confined. (Image: Max Planck Institute for Plasma Physics, Germany)
The first fusion reactions in a laboratory were achieved in 1934 — a major breakthrough at the time. Today, however, achieving a fusion reaction is not particularly difficult: in 2018, a twelve-year-old entered the Guinness World Records as the youngest person to successfully create a fusion experiment at home.
Unfortunately, such experiments produce bursts lasting just fractions of seconds, and achieving and sustaining these fusion reactions for prolonged periods remains a major challenge. Only by developing a steady and reliable way of producing fusion power can fusion become a commercially viable energy source.
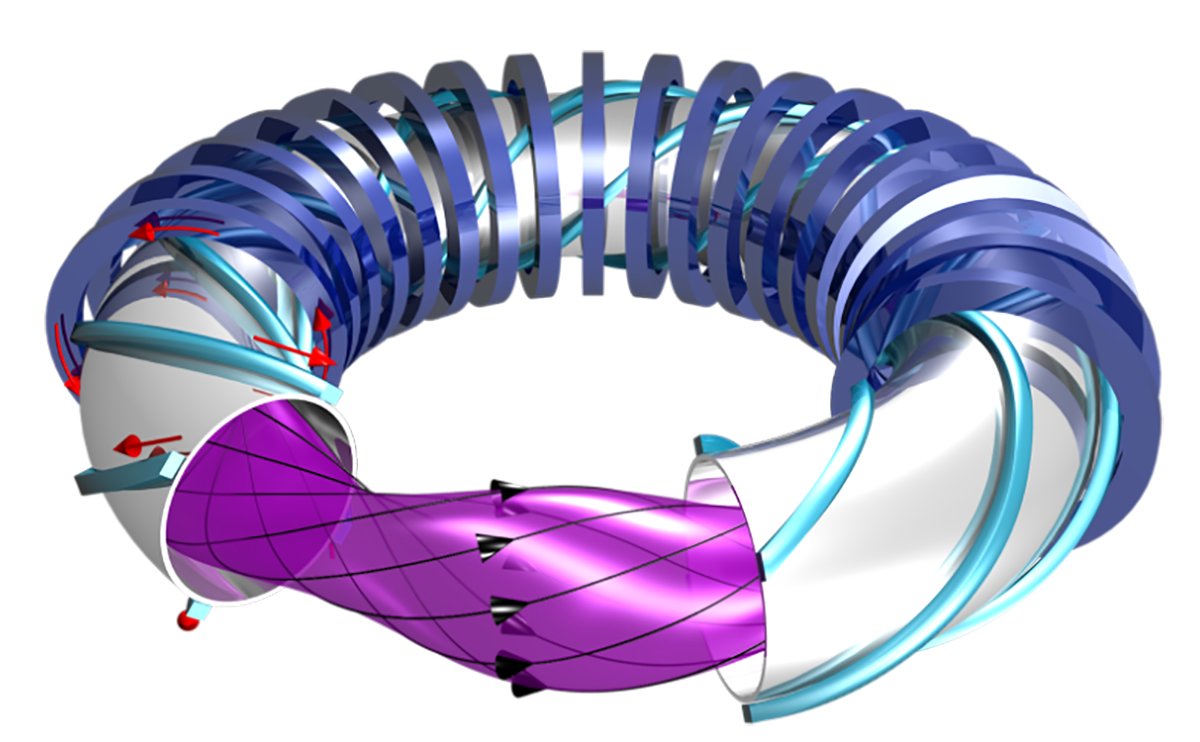
Twisting the magnets can also produce the helical shape without the need for a transformer — this kind of configuration is called a stellarator. (Image: Max Planck Institute for Plasma Physics, Germany)
Fusion power
Fusion power exploits energy released from the ‘fusion’ of light atomic nuclei. When two such particles merge, the resulting nucleus is slightly lighter than the sum of the originals. The difference, rather than disappearing, is converted into energy. Amazingly, this tiny loss of mass translates into a tremendous amount of energy that makes the pursuit of fusion energy highly worthwhile.
There are three states of matter: solid, liquid and gas. If a gas is subjected to very high temperatures, it becomes a plasma. In plasma, electrons are stripped from the atoms. An atom with no electrons orbiting around the nucleus is said to be ionized and is called an ion. As a result, plasma is made of ions and free electrons. In this state, scientists can stimulate ions so that they smash into one another, fuse and release energy.
Keeping plasmas stable in order to extract energy is difficult. They are chaotic, super-hot and prone to turbulence and other instabilities. Understanding, modelling and controlling plasma is extremely complex but researchers have made great strides over the past decades.
Scientists use magnetic confinement devices to manipulate plasmas. The most common fusion reactors of that kind are tokamaks and stellarators. Currently, these are the most promising concepts for future fusion energy plants.
Both reactor types make use of the fact that charged particles react to magnetic forces. Strong magnets in the reactors keep the ions confined. Electrons are also bound by the reactors’ forces and play a role in the surroundings. The magnetic forces constantly spin the particles around their doughnut-shaped reactor chambers to prevent them from escaping the plasma.
Same challenge, different solutions
As stellarator configurations are challenging to build, most fusion experiments today are tokamaks (a short form for a Russian expression that translates as ‘toroidal chamber with magnetic coils’). About 60 tokamaks and 10 stellarators are currently operating.
Both reactor types have certain advantages. While tokamaks are better at keeping plasmas hot, stellarators are better at keeping them stable. Despite the tokamak’s current prevalence, it is still possible that stellarators could one day become the preferred option for a prospective fusion energy plant.
Researchers have made great strides in magnetic confinement fusion and can now achieve plasmas of very high temperatures with ease. They have developed powerful magnets to handle plasmas and novel materials that can withstand the challenging conditions in the reactor vessels. Advances in experimentation, theory, modelling and simulation have led to a deeper understanding of the behaviour of plasmas, and devices like ITER will be central to proving the scientific and technical viability of fusion energy production.